Momentum Resolved Excitation Transmission Electron Microscope
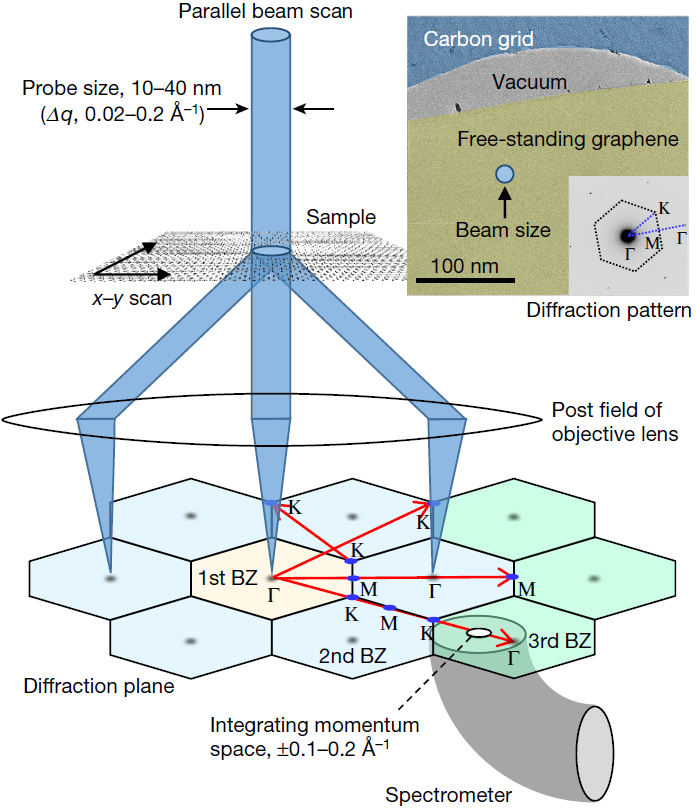
Momentum-resolved vibrational spectroscopy of graphite and hBN using EELS:
Fig.1: Schematic of the experimental set-up. Inset, a typical transmission electron microscopy (TEM) image of the sample and its diffraction pattern, showing a central spot. The EEL spectra are obtained from each spot along several lines, as shown in the lower part of the main panel, with the EELS aperture focused on the diffraction plane. The post field of the objective lens and other imaging lenses were used to form a magnified diffraction pattern.
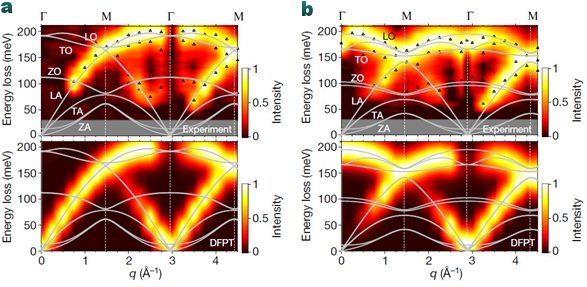
Fig.2: a, b, Color-coded intensity maps of graphite (a) and hBN (b) constructed from the measured EEL spectra (top) and from the simulated ones (bottom), shown with the simulated phonon dispersion curves (solid lines). Peak positions extracted from the measured spectra by line shape analysis are indicated by open triangles in the top panels. The grey regions show experimentally inaccessible energy regions where the peaks are difficult to discriminate from the quasi-elastic line. The observed redshift of the LO branch in the q = 0 Å−1 data can be explained by the suppression of LO/TO splitting occurring at the zone center in BN slabs of finite thickness, which is not taken into account in our present bulk calculation.
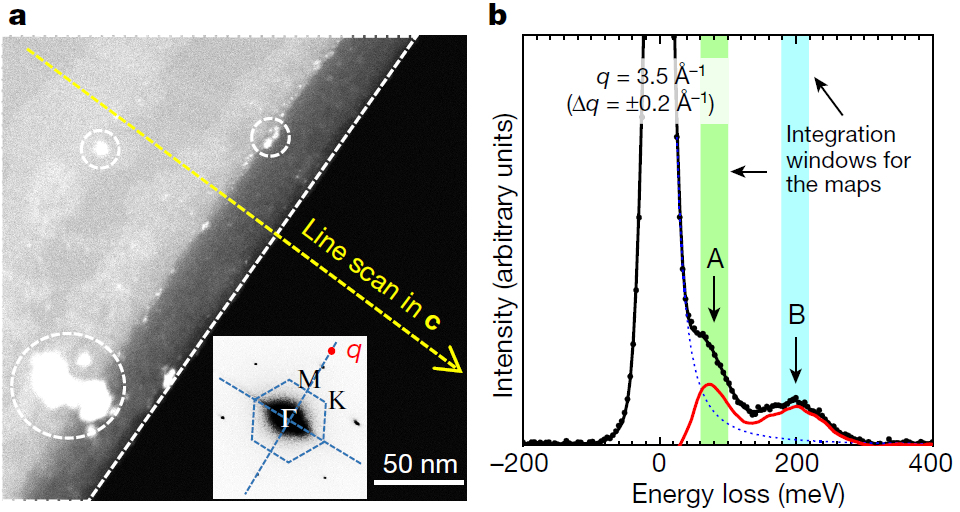
Position mapping of vibrations in graphene nanoribbons.
Fig.3: a, b Scanning transmission electron microscopy (STEM) image of a graphite edge with graphene nanoribbons on it; inset, its diffraction pattern. The dashed white line and circles indicate, respectively, the position of the edge and the positions of impurities. The red point in the inset indicates the location of the EELS aperture at q = 3.5 Å−1, where spectra were collected for the position mapping of vibrations. The direction of the measured q is parallel to the edge. The diffraction pattern suggests that the step edges are in the armchair direction 112¯0 . b, EELS spectra and integration windows for the lower-energy mode (A; green shading) that includes LA/ZO as well as edge and sp3 defect contributions, and for the higher-energy mode (B; blue shading) that includes LO/TO modes.
A major mission of condensed-matter physics is to understand material properties via the knowledge of the energy vs. momentum dispersion and lifetime of fundamental excitations. Unfortunately, the available techniques cannot be applied to emerging nanostructured materials. Inelastic x-ray scattering or electron energy loss spectroscopy (EELS) in reflection lacks the spatial resolution and EELS in transmission electron microscopy (TEM) lacks the needed energy and momentum resolution. In MORE-TEM, an innovative nano-spectrometer to measure momentum-dependent excitations with the unprecedented energy resolution (dE < 5 meV) should be developed. In addition, a temperature control down to liquid He, a simultaneously lateral resolution of tens of nm on samples as thin as an atomic monolayer are further goals of this project. In STEM mode an ultra-high spatial resolution should be achieved too.
This breakthrough should be realized by bringing together four groups with complementary skills ranging from TEM and electron optics to experimental/theoretical spectroscopy. MoRe-TEM opens the so-far unexplored possibility to investigate dispersion and lifetime of phonons, plasmons and excitons in hierarchical quantum matter including bio- and organic molecules, 1D nanotubes, 2D materials and van der Waals heterostructures, and nanocrystals of minerals and soft matter. Mapping out the energy and momentum landscape of primary excitations will make it possible to gain control on quantum phases, like charge-density waves and superconductivity, to engineer new materials for opto- and electronic nanodevices and for organic electronics, and to model the physical properties of natural geological systems. This will hugely impact a broad range of applications in the fields of solid-state physics, chemistry, biology, geology and engineering. MoRe-TEM not only implements features of a large synchrotron facility on a cheaper table-top instrument, but it also pushes the momentum-resolved spectroscopy to the realm of the nanoscale, providing thus a fundamentally new and unique infrastructure for the characterization of materials.
Tasks of CEOS
For CEOS this project means the development of a new monochromator at ground potential which allows the reduction of the energy width down to the requested energy width dE < 5 meV of the electron beam of a cold field emitter. One of the most important goals will be to optimize the electron gun in combination with the monochromator to achieve a beam current which allows the acquisition of EELS data with the requested precision. Besides the monochromator an ultra-high energy resolution spectrometer will be a prerequisite too.
The Corresponding Principal Investigator #1:
Prof. Thomas Pichler, University of Vienna, Faculty of Physics
Principal Investigator #2:
Prof. Francesco Mauri, Università di Roma La Sapienza, Faculty of Physics
Principal Investigator #3:
Prof. Kazu Suenaga, AIST, Osaka, Japan
Principal Investigator #4:
Prof. Maximilian Haider, [CEOS GmbH](), Heidelberg
Figures by courtesy of K. Suenaga. The full paper was published by:
R. Senga, P. Barone, S. Moryshita, F. Mauri and T.Pichler, Nature Vol.573 (2019) 247 – 250, https://doi.org/10.1038/s41586-019-1477-8